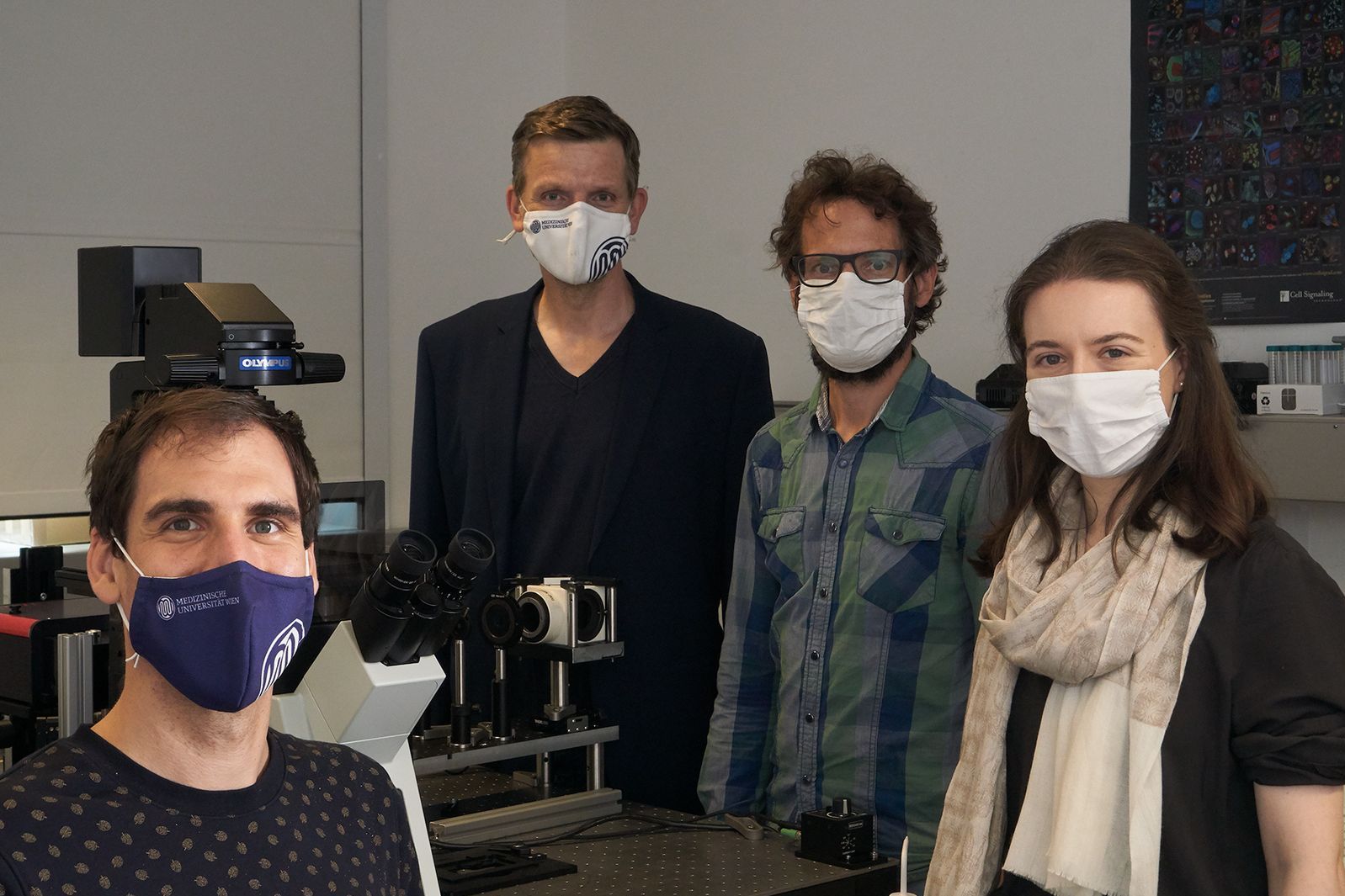
(Vienna, 09-10-2020) In fact, the technology contradicts a fundamental principle that had long been regarded as a natural law: light waves can only be used to scan objects that are larger than half the light wavelength. Although this rule is true, it can be outsmarted by illuminating different points of the object one after the other – for example very specific molecules on a cell. This is the basic idea behind super-resolution microscopy, for which the Nobel Prize in Chemistry was awarded in 2014.
Since then, this microscopy technique has proven itself time and time again all over the world but a team of scientists from Vienna University of Technology and MedUni Vienna has now shown that great care is needed in interpreting the data. Although super-resolution microscopy makes individual molecules visible it can easily happen that one molecule is scanned multiple times. A case of multiple exposure can then be confused with a cluster of multiple molecules. The research team developed methods for distinguishing between these two possibilities. These have now been published in the specialist journal "Nature Communications".
Seeing better than the laws of optics allow
If you make a mosaic from stones that are one square centimetre in size, you cannot depict structures that are only a millimetre in size. It is much the same in microscopy: if you scan a tiny little point, you obtain, at best, a light spot of the same size as the wavelength of the light you use. If you want to scan points that are very close together, they produce light spots that overlap each other, thus producing a blurred image.
"Super-resolution microscopy, as we use it, offers an elegant way out," explains biophysicist Mario Brameshube from the Institute of Applied Physics of Vienna University of Technology, who is one of the lead authors of the study. "One uses special molecules that are briefly excited by a laser beam, so that they light up. These molecules are then used to mark the structures one wishes to scan. If these molecules light up in sequence, each of them can be perceived as a diffuse disc of light – and the molecule has to sit more are less exactly in the centre of this disc of light." The position of the individual molecules can then be derived from multiple individual images and these can be computationally reconstructed to produce one high-resolution image.
One or many?
However, one encounters a problem when one tries to make a detailed examination of T cells, which play a major role in our immune system. "From both a biological and immunological point, it is important to know whether certain receptors, such as T cell antigen receptors, are evenly distributed over the surface of the cell or whether they are grouped together in so-called clusters," explains Johannes Huppa, biochemist and immunologist from MedUni Vienna’s Institute of Hygiene and Applied Immunology. "And as we found, it is precisely this question that is difficult to answer with super-resolution microscopy."
In fact, the team found that various molecules used as tracer dyes in super-resolution microscopy are able to light up not once but multiple times – in some cases up to 20 to 30 times. "And then one will draw completely false conclusions using conventional methods of analysis: it would look as if there were a cluster of 20 to 30 on the smallest area," says Huppa.
The statistics of light flashes
With a lot of effort and special biochemical tricks, the research team has now managed to accurately characterise various tracer dyes: special proteins were marked with various tracer molecules and then fixed on a glass plate. The behaviour of the molecules was then analysed in detail: do they light up multiple times when they are excited by a laser or do they disintegrate after the first illumination? How long does it typically take for them to light up again after the first time?
"With this information we can now simulate the behaviour of the molecules on a computer and compare it with super-resolution images from experiments," says Brameshuber. "And our simulations show certain statistical correlations between the light signals, which tell us whether individual molecules have delivered multiple signals or whether multiple molecules have each delivered a single signal in the experiment." The final image that is produced might well look the same to the naked eye but, using mathematics, it is possible to derive additional important information that improves the predictive reliability of the method, and makes it more robust.
"Super-resolution microscopy is now an extremely important technology and will continue to be so," agree the study’s two lead authors from MedUni Vienna and Vienna University of Technology, René Platzer and Benedikt Rossboth. "Our research project produced two important findings: firstly, that the interpretation of images is sometimes more complicated than previously assumed and, secondly that, with very careful experiments and calculations, one can nonetheless eventually obtain the information necessary for reaching reliable conclusions."